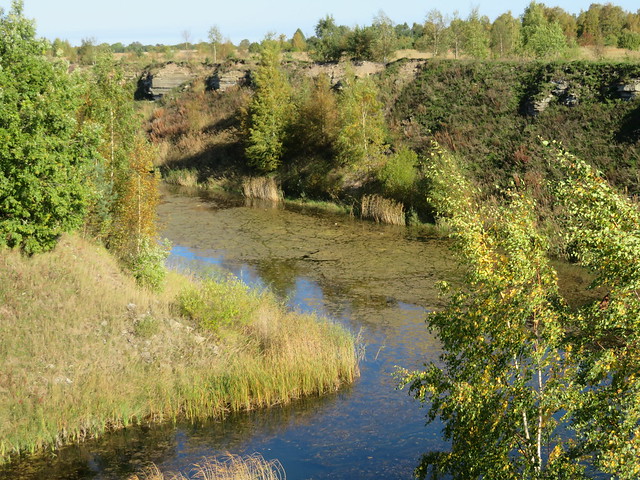
http://www.minest.ee/water
txt: GEOTECHNICAL PROCESSES AND SOIL-WATER MOVEMENT WITH TRANSPORT OF POLLUTANTS IN THE ESTONIAN OIL SHALE MINING AREA Tallinn Technical University, Department of Mining 82, Kopli Str., 10412 Tallinn, Estonia Abstract One of the most important industries of Northeast Estonia is oil shale mining. Ground movements caused by mining reach the ground surface easily due to shallow location of workings. A new, artificial topography is formed on undermined areas, where the ground surface depressions are alternating with rising grounds. When the Quaternary cover contains loamy sediments, the surface water will accumulate in the depressions. The response of usable lands on undermined areas depends on the degree of changes in the relief and water regime. The accumulation of solid residues by oil shale mines and processing plants has resulted in numerous ash hills, which are polluting the environment. The streams are polluted by phenols, oil products and sulphates. The main source of water supply is groundwater in the oil shale basin. The hydrostratigraphic section is represented by three aquifers. Two (Quaternary and Ordovician) of these aquifers are affected by the human activity. Intensive water consumption has caused a fall in the water level in these aquifers. Due to oil shale production the concentration of Ca2+, Mg2+, SO4 2- and Cl contained in the groundwater from the Ordovician deposits is noticeably higher than in the water with a natural background level. The natural water chemical regime is restored at the cessation of mining. Introduction Estonia is situated on the shore of the Gulf of Finland (Fig. 1) and is characterised by flat topography with only slight differences in elevation. Gulf of Finland Estonia Russia 20 kilometers 0 10 Narva Sirgala Estonia Kohtla - Järve ash dumps Kiviõli ash dumps Kohtla-Järve Sompa Tammiku Ahtme Kukruse Viru Sillamäe Mined out area Claims of oil shale mines Ash dumps Oil Shale outcrop ESTONIA LUXEMBOURG IRELAND DENMARK ANDORRA MONACO LIECHTENSTEIN BOSNIA AND HERZEGOVINA FR. YUGO. REPUB. OF MACEDONIA ARMENIA ICELAND LATVIA MOLDOVA LITHUANIA SLOVENIA PORTUGAL NETHERLANDS FRANCE SPAIN FINLAND NORWAY SWEDEN GREECE ROMANIA UKRAINE SLOVAKIA CZECH REPUBLIC POLAND BELARUS RUSSIA GEORGIA MALTA Fig. 1 Location map of Estonian oil shale deposit. Oil shale reserves are concentrated in North-East Estonia (Fig. 1) and make up about 3,8 billion tons, half of which can be mined according to existing technology, economical and ecological criteria. The annually exploitable layers have an average thickness of 2,6 m and the bedding depth increasing in the southern direction. Oil shale consists of 30-40% organic (kerogen) and 60-70% mineral matter (mainly carbonates and sandy-clayey minerals). The sulphur content averages 1,6 %, the net calorific value varies from 6-10 MJ/kg. Oil shale output increased from 2 million tons in 1940 to 31 million t in 1980, and has since decreased to 12 million tons (Fig. 2). 0 5 10 15 20 25 30 35 1920 1940 1960 1980 2000 2020 Output, Mt per year Output Forecast Fig. 2 Estonian oil shale annual production (mining output) and forecast by Enno Reinsalu (Reinsalu, 1998) The study object was of 290 km2 underground and 130 km2 strip mined area (Valgma, 2002). Oil shale is presently excavated in two surface and two underground mines. For excavation oil shale the mines must be dewatering. The mine water pumped up the oil shale underground and surface mines and discharged into rivers forms on the average 86% (quantitatively 159- 226 millions m3 /yr). In the region of oil shale mining on an average 15 million m3 of water is being pumped out of mines monthly (Fig. 3). Mines drainage causes the depletion of ground- 0 5 10 15 20 25 30 35 40 45 January February March April May June July August September October November December million m3/month 1999 2000 2001 2002 Fig. 3 Water, which pumped out of surface and underground mines monthly water, resulting in drawdown of 20 m in the northern and 70 m in the southern part of mining area. The infiltration of contaminants grows and the main aquifers are chemically polluted over a large area. The formation of the chemical composition of water is influenced by considerable variation of hydraulic conductivity, numerous faults in the strata, karst and, in addition, depressions forming during mining. Evidently each singular case represents a plurality of underlying causes and hence a considerable variety of analytical results depending on the location and season of sampling. Methods During years 1998...2001 geotechnical processes of closed underground oil shale mines and opencasts were investigated. A digital map of Estonian oil shale mining was created for joining data of technological, environmental, and social limitations in the deposit. The main objective was the stability of underground mined area. The stability was studied with help of aerial photographs, mine drawings, maps of quaternary sediments and mathematical modelling of rock failure. Water sampling took place during the spring and summer from 1988 to 1990. This period samples were from tectonic faults and karst fissures in mines, and also from pumped public supply or private boreholes. Springs and rivers were also sampled to obtain a reasonably complete hydrochemical understanding of the hydrogeological cycle in this area. The chemical analysis from more than 20 constituents was carried out on water samples from each of the 31 sampling sites. From Eesti Põlevkivi Ltd. and Estonian Geological Survey were received the official data for the period 1991-2002 and these are following: annual amounts of mine waters, chemical content of water, annual influxes of sulphates with mine waters, data of water regime, the chemical content of ash dumps water. Results The main influences of underground mining on the surface are spontaneous collapses and subsidences. Subsided land is located above hand-mined, advancing-and-retreating mining and longwall mining with double-unit-face areas. The relief of subsided land depends on the quantity of the filling material and filling quality, and on roof structure. Information was collected mainly about random spontaneous collapses of drifts and spontaneous collapses of room-and-pillar mining. Some information is collected about induced caving of longwall mining in Kohtla mine. Considering that longwall mining was stopped in the Estonian deposit in the year 2001, the information about induced caving should be collected and analysed as well. The area of subsidence moulds is in average 55600 m2 ranging from 2500 to 152500 m2 in total. There are 3.1 km2 subsidence areas. Some of the spontaneous collapses mentioned by mining surveyors cannot be recognized on the surface and some seen on the surface have not been reported. In these cases the fieldwork was done with GPS device and caving possibilities considered with the help of digital maps. Cavings have been found in the areas where mines operated within the period of 1963...1989. Surface mining mapping included digitising haulage and mining trenches, loading points, dewatering constructions like ditches, basins and tunnels. Besides, yearly mining ranges were digitised. Created database includes geological characteristics and data of the used technology. Map of yearly mining ranges offers a good overview of the mining period, speed and extent. The present open-cast landscapes can be divided into four classes. The first one is the afforested (mainly with pines) area. The second one is the area with poor vegetation, small trees and bushes, fifty per cent of it being a rocky surface. The third one is a graded area, mainly without vegetation but ready for planting. The fourth area has spoils that are not graded and have no vegetation; their surface angles are reaching the angle of the repose, maximum 45o. One year stripping equals 1-3 trenches. As an example, the former Narva open-cast has been modelled. Digital elevation model (DEM) was created from geological, topological and mining data. There are two principal types of spoils in open cast mined areas. First is formed by beginning mining technology in 1925 to 1950. Oil shale layers were mined by handwork and overburden was stripped by 3 m3 steam excavator in cooperation with spreader. The width of the mining pit was 20 m, forming spoils with maximum slopes 20o. The thickness of the oil shale layer was 2.6 m and the overburden thickness varied from 5 to 12 m. Average stripping factor was 1.9 m3 t-1. Due to this, average height of the ground in the mined out area is from 0 to 2 m below original ground surface, forming deeper and higher peaks. Depending on that, the moisture content of spoil might be higher than that of the soil in the same area before mining. Since spreader and mechanical shovel were used for stripping, the estimated swell factor of the material was 1.3. Maximum size of the material could be 1.4 m due to bucket size and average size was 150 mm. Because of low mobility of the spreader and missing reclamation requirements, the unevenness of the spoil varies between 0.5 and 2 m. The content of organic matter in the spoil, originating from kukersite oil shale, is on average 4%–6%. That material is evenly spaced in the spoils due to stripping method. The second type is mined with newer technology beginning from 1950ties. The area was mainly covered with swampy forests and bogs. Oil shale layers were mined by blasting and overburden was blasted and stripped by 15 m3 and 90 m long boom draglines. The width of the mining pit was 50 m, forming spoils with maximum slopes 3o. The thickness of the oil shale layer was 2.0 m and the overburden thickness varied from 11 to 17 m. Average stripping factor was 4.1 m3 t-1. Due to this, average height of the ground in the mined out area is about 2 to 4 m above the original ground surface. Depending on higher surface than natural and water pumping due to operating open cast, the moisture content and seepage of the spoil might be lower than in the first area. Organic part of the left oil shale layers is mostly in bottom. Dragline and in some cases mechanical shovel were used for stripping of blasted overburden rock, therefore the swell factor of the material was 1.4. Maximum size of the material is 3.1 m due to bucket size and average size was 300 mm. Unevenness of the spoil ranges from 0.5 to 1.5 m on average, but it can be up to 6 m in test sections. The content of organic matter in the structure of spoils, originating from kukersite oil shale, is on average 2% being 4% in bottom and 1% in surface of the spoil. Due to stripping method the material is unevenly spaced in the spoils, the estimated swell factor is up to 1.5 in the bottom. This is another reason for water runoff from the spoils. Because of several mines are being closed during next few years the problems of drowned waste are going to be more actual than before: increase of underground water level, underground water pollution, technogenic water sources and over flooding of reclaimed areas. The chemical analyses of groundwater divide into two principal groups (Fig. 4): - a very large group of CaHCO3 waters; - a large group of Mg(Ca)SO4(HCO3) waters. 0 100 200 300 400 500 600 700 mg/l underlying 7 4 67 17 309 10 10 overlying 10 9 59 30 356 10 9 closed mine (Tammiku, Sompa, Kukruse, Ahtme) 30 7 181 47 385 370 36 working mines (Estonia, Viru) 20 10 156 50 427 620 57 surface mines (Sirgala, Narva) 12 8 174 55 353 530 35 Na K Ca Mg HCO3 SO4 Cl Figure 4. Chemical compositions of groundwater and mining water The influence of groundwater consumption, dewatering of surface and underground mines on the groundwater regime was evident. In the course of oil shale mining at the Estonian oil shale deposit three stages of Ordovician aquifer system have been partially or totally drained. The observation data show that all the aquifers are interconnected and oil shale mining exerts a remarkable influence on all of them. Contamination in this region is mostly due to pollution caused by power and chemical industries. Studies of ash hills demonstrate that the ash may be highly enriched in certain potentially toxic elements, typically semi-volatiles, such as boron, arsenic, antimony etc. The ash dumps water (Table 1) is typically rich in oxides and may have an extremely high (alkaline) pH, which may render some metallic or semi-metallic species highly mobile. Table 1. The composition of the ash dumps water Content, g/m3 Pollutants Interval Maximum Chemical oxygen demand (COD) 4000-8000 18000 Biochemical oxygen demand (BOD) 2500-4000 6400 Phenols 200-580 1400 Volatile phenols 80-110 150 Oil products 30-70 100 Benzo(a)pyrene, g kg-1 0.05-0.15 0.22 Sulphides 100-230 270 Dry matter 3500-6000 7300 Suspended solids 300-600 1300 Total hardness, eqv m-3 30-47 53 pH 11.4-12.2 12.5 There is increasing evidence that portions of the water infiltrating through the soil surface may move rapidly through the aeration zone along preferred flow paths such as macropores and fractures. This rapid, concentrated flow may also have significant implications for the transport of pollutants to the groundwater body. Discharge into the macropores and the concentrated groundwater recharge in the vicinity of the macropores occurred in relation to the development of the groundwater mound. Incorporation of the soil-water movement would bring better prediction of the time, location and magnitude of groundwater pollution due to the transport of pollutants by the infiltrating water. Discussion The basic processes that determine the chemical composition of water in the samples can be classified as follows: - chemical composition of water in the structurally undamaged aquifers; - chemical processes taking place in the sites of tectonic damage and erosion; - infiltration of groundwater (atmospheric precipitations and extracted water) through loading layers; - man-made changes in the environment. As a result of the combined influence of these processes the natural bicarbonate-type water, characteristic of the Ordovician aquifer of the given region, has changed into sulphate- bicarbonate water, having increased from 0,2...0,3 g to 1,0 g/l. Essential role in the formation of the chemical composition of water, especially in shaping local singularities, is played by tectonic damages and karst; in that case the value of the hydraulic conductivity increases. In the regions where tectonic faults are widespread general mineralization may be twice as high as that usually characteristic of water aquifer. Concentrations of Na+ , Ca2+ and Cl are also much higher. Very significant part in the formation of the chemical composition of waters is played by depressions that have sprung up in the course of mining. Their impact is two-hold: infiltration and water exchange increase significantly and with the alteration of aeration conditions a geochemical environment with new physical-chemical properties is formed. Conclusions Natural hydrogeological conditions in Northeast Estonia were simple, but they have been disturbed by the mining industry and consumption of the groundwater. Deeply fractured carbonates, together with effects of mining, have facilitated the rapid spread of aquatic pollution. Mineralised mine water extraction is useless, and the reduction of its mineralization level by technological means or natural filter systems is a problem which needs tackling in the near future. Today this water is guided to natural water bodies. As the content of sulphates in mining waters is high, the concentration of sulphates in the bottom sediments of many water bodies has risen sharply. Due to strong eutrophication there is an oxygen deficiency in some places and the reduction of sulphates to toxic H2S already occurs. The study was supported by EstSF GRANTs G3403 and G4870. Bibliography 1. Reinsalu, E. Is Estonian oil shale beneficial in future? Oil Shale, 15/2, 1998, 97-101. 2. Valgma, I. Estonian oil shale resources calculated by GIS-method. Symposium on Oil Shale., Tallinn, Estonia, 18-21 November 2002